Researchers seek universal treatments to impede coronavirus
New research from a team of Cornell collaborators points to a possible target for antiviral treatment for COVID-19.
Their review paper, “Coronavirus Membrane Fusion Mechanism Offers a Potential Target for Antiviral Development,” published April 6 in Antiviral Research. The multidisciplinary group was led by Susan Daniel, associate professor of chemical and biomolecular engineering, and Gary Whittaker, professor of virology at the College of Veterinary Medicine.
The researchers initially set out to analyze the structure and characteristics of SARS-CoV (severe acute respiratory syndrome coronavirus) and MERS-CoV (Middle East respiratory syndrome coronavirus), with a focus on the spike protein – specifically the fusion peptide – that allows these viruses to infect cells by transferring their genome.
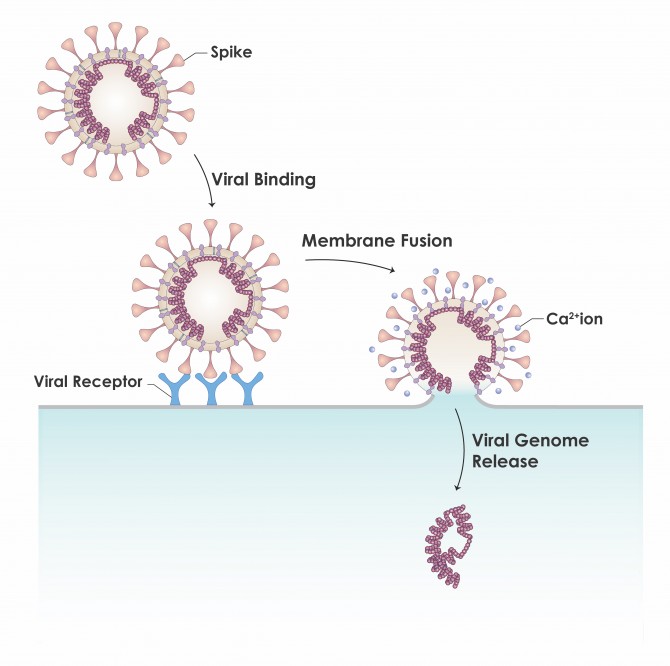
The coronavirus attaches to the host cell by way of the spike protein, which binds to a specific receptor on the host cell’s surface. The spike protein also contains parts that facilitate the fusion of the viral membrane with the host cell membrane to deliver the viral genome inside the cell. Calcium ions have been implicated in promoting this membrane fusion step through interactions with the fusion machinery.
As the current pandemic escalated, the researchers compared the biological sequences of the fusion peptides of SARS-CoV to SARS-CoV-2, the virus that causes COVID-19, and found them to be a 93% match.
“What’s really interesting about SARS-CoV and MERS-CoV, and this new virus, SARS-CoV-2, is this particular part of the protein, the fusion peptide, is almost exactly the same in those three viruses,” Daniel said. “Blocking the fusion step is significant because the fusion machinery doesn’t evolve and change as fast as other parts of the protein does. It’s been built to do a particular thing, which is to merge these two membranes together. So if you can develop antiviral strategies to reduce that efficiency, you could have potentially very broadly-acting treatments.”
Daniel’s lab specializes in the biophysics of the cell membrane envelope; Whittaker’s team studies the entry of influenza and coronaviruses into host cells. Their combined group is drilling down into the intricate procedure of membrane fusion – a critical part of the mechanism by which coronaviruses spread.
Membrane fusion is a multistep process that begins with the virus recognizing that it’s found the right type of cell to infect. To do this, the virus receives feedback from the chemical environment, including cues like the receptor that the host cell presents. The virus then attaches to the host cell receptor by way of the spike protein.
Next, a piece of the spike protein, called the fusion peptide, interacts directly with the host cell membrane and facilitates merging to form a fusion pore, or opening. The virus then transfers its genome into the host cell through this pore. These genomic instructions essentially commandeer the host’s machinery to produce more viruses.
These interactions can be difficult to parse through traditional approaches because the fusion process is dynamic and flexible, and the spike protein changes its shape drastically during fusion. So Daniel and Whittaker turned to Jack Freed, the Frank and Robert Laughlin Professor of Physical Chemistry Emeritus, and David Eliezer, professor of biochemistry at Weill Cornell Medicine, who used a variety of spectroscopic methods including electron spin resonance and nuclear magnetic resonance, respectively, to more closely analyze the fusion peptide’s structure and function.
The group found that calcium ions interacting with the fusion peptide can change the peptide’s structure, and how it interacts with membranes in ways that promote infection in MERS and SARS. Now, the researchers are turning their attention to SARS-CoV-2 because the fusion peptides are consistent in all three viruses.
The team is hopeful the research can illuminate some of the chemistry-related questions surrounding COVID-19, such as how it was able to move into humans, what chemical cues facilitated that process, and why the virus is able to replicate so easily in the respiratory tract.
“The subtleties are what make this virus so interesting,” Daniel said. “For some viruses, calcium has no effect on infection, but for coronavirus, we are seeing that it does.
“And this points us to thinking about how this might be a target for developing antiviral drugs or repurposing already approved calcium-blocking drugs,” she said. “Based on what we know, we believe that targeting the fusion machinery for drug development is an astute choice.”
The team’s findings have led to supplemental funding from the National Institutes of Health (NIH), through its Research Project Grant program, to develop an antibody that could block the virus’s entry by interacting with the fusion peptide. And an adjacent collaboration between Daniel, Whittaker and Nicholas Abbott, a Tisch University Professor in the Robert F. Smith School of Chemical and Biomolecular Engineering, was recently awarded National Science Foundation (NSF) Rapid Response Research funding to explore additional properties of the fusion peptide, which could help researchers predict what cells are likely to get infected.
“The part of the infection cycle we are studying is a conserved process. The virus is always going to need to transfer its genome,” Daniel said. “But it is challenging. The reason we don’t have good antivirals that can stop fusion is because it is a difficult region to target. What’s exciting about our work is we’re providing insight into how all this machinery works, which is essential for the development of new antivirals.”
The paper’s lead author is doctoral student Tiffany Tang. Doctoral student Miya Bidon and postdoctoral researcher Javier A. Jaimes are contributing authors.
The research was supported by the NIH and NSF.
By David Nutt