Science alliance: National collaborations strengthen CVM impact
Researchers at the College of Veterinary Medicine investigate complex problems affecting animal, human and public health. These issues involve biological, environmental, economic and social factors and have implications that reach far beyond New York state. To grapple with these multifaceted questions, CVM researchers forge national collaborations to further the scope and impact of their work.
Fighting tick-borne disease in the Northeast
In her innovative research to develop new veterinary diagnostic techniques, Laura Goodman, Ph.D. '07, assistant research professor in the Department of Population Medicine and Diagnostic Sciences, is one of several CVM faculty members who collaborate with the Northeast Regional Center for Excellence in Vector-borne Diseases (NEVBD). This group of academic and public health professionals come from 60 institutions across 13 states in the Northeast United States, from Virginia to Maine, including the Bloomberg School of Public Health at Johns Hopkins University, the Earth Institute at Columbia University and the Maine Medical Center Research Institute.
“A lot of times, when academics say ‘collaboration,’ we mean with other academics, but my group and NEVBD are well-situated to work with public health and industry,” says Goodman. “We really try to engage with other stakeholders and leverage big data for the greater good.”
We try to understand that this is not only a biological question. It's almost a philosophical question, because there will be economic winners and losers.
- Dr. Yrjö Gröhn, James Law Professor of Epidemiology
NEVBD members conduct applied research on tick-borne diseases, train scientists and health practitioners and cultivate a community of people who address these issues. “People have been working in silos on these problems, so what we’re trying to do is bring everyone together and enhance collaboration,” says program director Dr. Laura Harrington, professor of entomology in the Cornell University College of Agriculture and Life Sciences.
Goodman oversees diagnostic research and development for NEVBD partner institutions at the Animal Health Diagnostic Center/New York State Veterinary Diagnostic Laboratory (AHDC/NYSDVL). Collaborating with the group increases her ability to track tick-borne diseases in New York, to perform testing in partner states and to discover emerging diseases in the Northeast. In 2018, she was the first to detect the tick-borne parasite that causes babesiosis in Tompkins County. The parasite is gradually moving north through the state.
Collaborating with NEVBD also raises the profile and scale of Goodman’s work so that she is more competitive for national grants. Currently she is looking for support to defray the cost of testing ticks submitted to the Cornell Tick Evaluation Program. This program, which identifies a tick’s species and screens it for disease, has been offered for 10 years. Historically, veterinary practices have submitted the majority of samples, but last year Goodman opened the service to the public.
In 2017, the AHDC clinical molecular diagnostics and parasitology teams, directed by Amy Glaser, D.V.M. '87, Ph.D. '95, and senior extension associate Dr. Manigandan Lejeune Virapin, tested almost 700 ticks from pets, people, livestock and property. And they expect those numbers will only go up. Reports of diseases from tick, flea and mosquito bites tripled in the United States between 2004 and 2016, and nine new types of infections have been detected in that time, according to the Centers for Disease Control and Prevention (CDC).
Fortunately, Goodman has developed a “tick chip” — a nanoscale PCR testing platform for rapid detection of tick-borne diseases. In 2017, the American Association of Veterinary Laboratory Diagnosticians awarded her the first ever Innovation in Veterinary Diagnostic Medicine Grant for this technology. Each chip can detect up to 26 disease agents from 48 samples in a single day.
“Several states have contacted Dr. Goodman to see about having their tick samples tested at her lab,” says NEVBD program manager Emily Mader. “Few labs can run this volume of samples, with a quick turnaround time and tick identification.”
In collaboration with NEVBD, Goodman and Lejeune also work with students from the Cornell Master of Public Health program, and the new master of science in entomology program in vector-borne disease biology. Through these collaborations, Goodman not only expands the reach of her own work, but is helping train the next generation of diagnosticians who will tackle this pervasive problem.
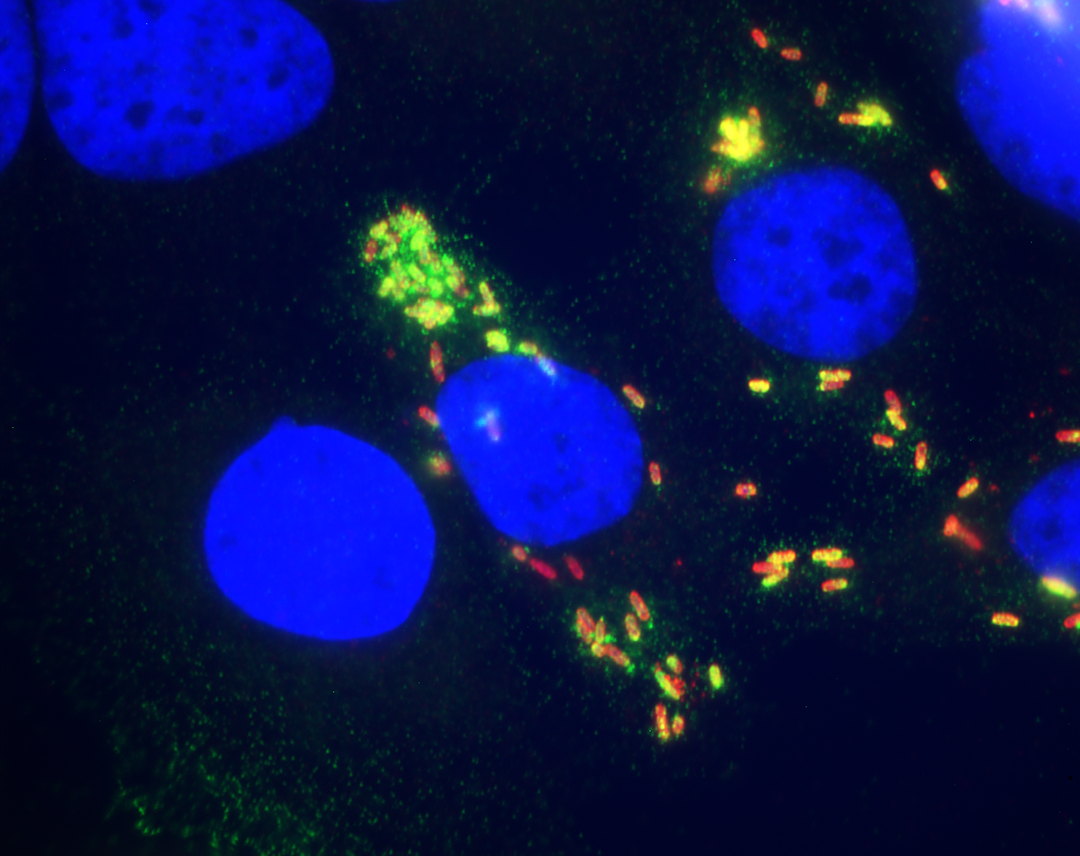
A federal investigation

CVM researchers also collaborate with federal agencies to inform policy decisions and tackle complex problems that require insight from multiple disciplines, such as the rise of antibiotic resistance. Prevailing assumptions contend that antibiotic use on farms accelerates the growth of bacteria that are immune to drug treatment, causing government agencies in many countries to impose limits on agricultural use.
When the U.S. Food and Drug Administration (FDA) began considering ways to limit the use of antibiotics in livestock — leading to a ban on antibiotics for growth promotion that went into effect in 2017 — Dr. Craig Lewis of the Veterinary Medical Office at the FDA approached Dr. Yrjö Gröhn, James Law Professor of Epidemiology. Lewis wanted to know if he could predict the impact of such a ban. Throughout his career, Gröhn has used mathematical modeling to understand how disease spreads on farms with the goal of improving food safety. “For me it almost doesn’t matter what is the problem,” says Gröhn. “I want to understand the whole food supply, from produce all the way to the consumer.”
Gröhn began collaborating with the National Institute for Mathematical and Biological Synthesis (NIMBios), uniting experts across disciplines to tackle the thorny question of agriculture’s role in creating antibiotic-resistant bacteria. He worked with fellow veterinary researchers, as well as experts in the FDA, the U.S. Department of Agriculture and the CDC to compile publicly available data regarding antibiotic usage and resistant organisms detected in meat and dairy products. The United States does not track individual farm animals, however, and much of the data is anonymous, making it impossible to connect data points from along the food supply chain.
To cope with this problem, the group focused on two types of data. They looked at antibacterial resistance detected at slaughterhouses as a way to represent the number of bacteria evolving resistance on farms, and at bacterial data from retail establishments to represent the human exposure to these resistant organisms. Using traditional statistical methods, graphical techniques and machine learning, they tried to identify trends between the two pools of data.
The group concluded that they didn’t yet have enough information to conclusively determine the relationship between antibiotics usage on farms and the rise of resistant bacteria. They made specific recommendations that are being followed for additional data collection regarding how farmers are using antibiotics in the production of cattle, poultry and pork, under existing laws. With this additional information, the group can make recommendations to inform FDA policies.
This project has since spun off into a new collaboration called “Living With Resistance,” through the National Socio-Environmental Synthesis Center (SESYNC). “We try to understand that this is not only a biological question,” says Gröhn. “It’s almost a philosophical question, because there will be economic winners and losers.” The collaboration includes plant scientists and social scientists and will address not just antibacterial resistance, but other types of drug resistance, as well as pesticide resistance in weeds and insects, and the spread of invasive species.
Coast-to-coast collaboration to stamp out Typhoid

National collaborations also give researchers access to specialized technology that may be available nowhere else in the country. Dr. Jeongmin Song, assistant professor of microbiology and immunology, started just such a collaboration to advance her research on how the bacterium responsible for typhoid fever establishes long-term infections that cause some patients, like the famous Typhoid Mary, to shed the bacterium for decades.
People usually contract the bacterium that causes typhoid, called Salmonella Typhi, through contaminated food or water, making it a problem primarily in resource-limited settings. The infection can be cured with antibiotics, but multi-drug resistant strains are becoming increasingly common in many areas. The FDA has approved two typhoid vaccines, but they are only about 70 percent effective. Even after vaccination, S. Typhi can slip past the immune system and hide out in white blood cells, called macrophages, as well as the gallbladder, to create a long-term infection. “The bottom line is that all of the available vaccines work well but they don’t show 100 percent efficacy,” says Song. “We need to develop alternative therapeutics and improve the vaccine.”
Song and her lab members focus on typhoid toxin, a virulence factor made by the bacterium. “If we target an essential virulence factor, then we have a very good chance to eradicate this pathogen,” says Song. This protein triggers the immediate symptoms of typhoid — lethargy, stupor, malaise, a drop in white blood cell counts and in rare cases, neurological complications — and is instrumental in establishing persistent infections. Not only does the toxin injure cells, but it also helps traffic S. Typhi to specific parts of the body.
Song teamed up with Dr. Ruth Nussinov, a senior investigator at the National Cancer Institute in Rockville, Maryland, and expert in computer simulation to model how typhoid toxin’s receptor binding subunit attaches to specific chains of sugars that stick out from the surface of the body’s cells, called glycans. Song also worked with Dr. James Paulson, a glycobiology expert at the Scripps Research Institute in La Jolla, California, whose group developed an array printed with different types of glycans. With this array, Song recently tested which types of glycans the toxin prefers to bind.
“We get so many requests to use our array, so we’re pretty careful about who we get involved with,” says Paulson. “But Dr. Song was very clear about what she was looking for and that she was able to provide us with samples that are biologically active in glycan binding, which made it an interesting, high-priority collaboration.”
If we target an essential virulence factor, then we have a very good chance to eradicate this pathogen.
- Dr. Jeongmin Song
The array data showed that the typhoid toxin formed stronger bonds when the glycan had multiple branches. This specific type of branched glycan primarily occurs on the surface of immune cells and on endothelial cells that line the arteries in the brain, providing an explanation for how typhoid toxin attacks these two cell types, which likely helps them establish a persistent infection.
Furthermore, when Song gave mice that are susceptible to typhoid toxin a vaccine against it, they were fully protected. Song thinks that a human vaccine that also targets the toxin would be more effective than existing vaccines. Such a vaccine may prevent long-term infections, as well as typhoid symptoms, so that we could one day eradicate typhoid.
A new twist on the lymph system
Collaborations can also advance a scientist’s research by letting her tap into the highly specific expertise of a colleague. These collaborations can be especially helpful when one’s research takes a sudden left turn due to some unexpected findings, which happened to Dr. Natasza Kurpios, associate professor in the Department of Molecular Medicine. Kurpios’ group studies how organs form, specifically, how tissues deviate from the body’s initial symmetry to create asymmetric organs, like the heart and stomach.

Asymmetry is especially important in the small intestine. As it grows and lengthens, it begins looping in the counter-clockwise direction so that it fits neatly into the gut cavity. A springy, elastic tissue called mesentery suspends the intestines inside the abdominal cavity. Mesentery is crisscrossed with blood vessels supporting the gut. If the small intestine loops in the wrong direction, it can kink like a garden hose and cut off its own blood flow. This can happen in babies with a rare birth defect, creating a potentially deadly pediatric emergency requiring surgery.
There’s a lot of lipid people, there’s a lot of liver people, and there’s a lot of developmental people, but there are very few who are working at the borders. That’s why these collaborations are so important.
- Dr. Natasza Kurpios
By studying development in embryonic chicks and mice, Kurpios’ lab has discovered that besides holding up the growing intestine, the mesentery also gives the signal that begins the process of counter-clockwise looping, thanks to a master regulator called Pitx2. Cells in the mesentery also diverge developmentally to become blood vessels that nourish the intestines, and lymphatic tissues that transport dietary fats. “We believe that Pitx2 sets the stage for asymmetric gut looping and downstream of this pathway is how blood vessels and lymphatics form,” says Kurpios. “If the gut rotates one way, the blood vessels and lymphatics have to follow.”
Further experiments showed that the lymphatic system in the gut depends on the genetic program that makes arteries in the mesentery. This finding overturned a century-old idea that all lymphatic tissues derive from veins. The discovery also plunged Kurpios into the unfamiliar world of the lymphatic system.
Lining the intestine are finger-like projections called villi that absorb nutrients. A lymphatic vessel called a lacteal pokes into each villus to transport most fatty acids from the diet. Healthy lacteals transport these fats to the bloodstream via the lymphatic system, but when lacteals fail, they cause non-alcoholic fatty liver disease, obesity and problems with trafficking white blood cells.
To further investigate how Pitx2 controls lymph tissue development, Kurpios’ group obtained a mouse in which the Pitx2 gene is barely active. Due to an unknown malfunction, the mice developed fatty liver disease because the fats travelled straight to the liver, rather than the lymphatic system. Its lymphatic collectors looked clogged, like “beads on a string” and it had symptoms similar to a rare disease that affects humans and some dog breeds, called intestinal lymphangiectasia.
Kurpios had a fascinating metabolic problem on her hands, so she consulted with Dr. Patrick Tso, director of the Cincinnati Mouse Metabolic Phenotyping Center. “Patrick Tso is a pioneer in lipid metabolism and he has been a big supporter of our research,” says Kurpios. “He has been able to provide us with reagents and guidance to look at fat metabolism, so we can figure out how Pitx2 is regulating lymphatic function in these mice.”
Kurpios plans to visit Tso’s lab so that she can run diagnostics on her sick mice. “There’s a lot of lipid people, there’s a lot of liver people, and there’s a lot of developmental people, but there are very few who are working at the borders,” says Kurpios. “That’s why these collaborations are so important.”

By Patricia Waldron